Chapter 10
What can we do?
Broadly speaking, there are three strategies: Reduce emissions of greenhouse gases, compensate for them through climate engineering, and adapt to climate change.
10a Introduction
Which solutions we deploy to deal with climate change is not decided by scientists, engineers, or economists—it’s decided by society as a whole. After we understand the climate risks and the options for dealing with them, it’s up to us to choose how much risk we’re willing to assume on behalf of future generations, and therefore which actions to take.
This is a terrifically difficult decision because the costs of action may be high and those paying them are not likely to reap the benefits themselves. Indeed, there are few historical examples of civilizations consciously making sacrifices on behalf of descendants two or more generations removed. Yet, if we are to stave off the worst impacts of climate change, this generation must decide what actions it will take, and the following chapter briefly introduces the kinds of large-scale technological solutions that are available to us.
Options for dealing with climate change fall into three broad categories: curtailing the emissions of greenhouse gases and/or taking greenhouse gases out of the atmosphere (mitigation), learning to live with the consequences (adaptation), and engineering our way around the problems that greenhouse gases produce (geoengineering).
10b Curtailing emissions
Of these three options, mitigation has the most straightforward effect on climate because it attacks the source of the problem. Some aspects of mitigation might be worth undertaking anyway. For example, consumers might spend extra money on a high-efficiency car if the excess cost is paid back in fuel cost savings over a few years. Similarly, the costs of constructing or retrofitting buildings to conserve energy might also be paid back in a short time. Such conservation measures would not only help reduce emissions but would also prove economically beneficial for consumers.
The global problems of climate, energy, poverty and population are inextricably linked.
But given the actual and expected growth in the economies of developing nations such as China and India, conservation alone cannot begin to reduce greenhouse gas emissions to safe levels. Experience has shown unequivocally that rapid economic growth can only be achieved with large increases in per capita energy consumption.
The alleviation of the wrenching poverty of poor nations is, of course, a highly desirable goal, and one that the world has made impressive strides on in large part because modern electricity, heat, and transportation have been extended to more and more of the global population. To deny the benefits of energy growth to developing nations would be counter to one of the major goals of curtailing climate change: to assure a more prosperous future for humankind. Thus the challenge of mitigation is not just to decarbonize our current energy system, but to deliver decarbonized energy at an even greater scale than today’s fossil fuel-based system.
Experience also shows that as people are lifted out of poverty, they reliably choose to have fewer children—lowering the rate at which our energy demand increases in the future. In this way global trends in climate, energy, poverty and population are inextricably linked.
The growth in solar and wind power in recent decades has been truly impressive.
And the price of these energy sources has fallen as demand increases and technology improves.
At the present rate of consumption, oil and gas reserves are projected to be exhausted by late in this century, and coal early in the next. Thus in the not-too-distant future fossil fuels will have to be replaced anyway. Fortunately, the means of decarbonizing energy are at hand. The growth in solar and wind power in recent decades has been truly impressive, and the price of these energy sources has fallen as demand increases and technology improves. Even so, solar and wind provide only 8% of global electrical power today, and most energy experts believe that the Solar generators only produce power when the sun is shining, and wind turbines only spin when it is windy, for example. In other words, these sources provide power that is not continuous because it is tied to changeable natural processes.inherent intermittency of these sources will limit their market penetration, barring a true breakthrough in energy storage and/or transmission technologies.
Nuclear fission provides about 10% of global electrical energy, but today relies entirely on light-water reactors that produce radioactive waste. Even so, nuclear fission is far and away the safest form of energy mankind has ever produced. The mortality per kilowatt hour generated is less than that of most other energy sources, and comparable to solar and wind. While much is made of events such as that at the Fukushima facility in Japan, petrochemical accidents brought about by the earthquake and tsunami killed many while no deaths resulted from Fukushima’s release of radioactive material. Indeed, it is estimated that nuclear fission has saved about 1.8 million lives by displacing fossil fuels, whose combustion is the source of numerous health problems.
Nuclear fission is the safest form of energy humans have ever produced.
The mortality per kilowatt hour generated is less than that of most other energy sources, and comparable to solar and wind.
Yet nuclear technology has advanced significantly since light-water reactors were introduced more than a half-century ago. Advanced reactors operate at ambient pressure and are passively safe, so they are inherently incapable of melting down. They burn fuel far more efficiently, resulting in greater power production per unit input of fuel, and much less radioactive waste. They are far more environmentally benign than solar or wind, requiring much less land, and some of the new designs require very little water for cooling.
Actual experience in countries such as Sweden and France shows that fission power can be ramped up to supply a large fraction of electrical energy in less than 15 years. What is now lacking more than anything else is political will.
The implementation of nuclear fission, higher efficiency vehicles and buildings, and other mitigation measures could be accelerated by a variety of governmental actions, such as carbon taxes, cap-and-trade policies, subsidies for carbon-free energy, and removing existing subsidies for the coal, oil, and natural gas industries. While it is beyond the scope of this primer to discuss policy, it is clear that these approaches could greatly accelerate the implementation of these climate mitigation solutions.
Learn More
- MIT Explainers on Low-Carbon Energy Sources
- TILclimate Podcast: Today I learned about fossil fuels
- TILclimate Podcast: Today I learned about wind and solar power
- TILclimate Podcast: Today I learned about nuclear power
- MIT Energy Initiative Report: The Future of Nuclear Energy in a Carbon-Constrained World
10c Removing carbon
Another mitigation strategy is to reduce the effect of emissions by capturing and storing their greenhouse gas components. Such technology exists today and is considered necessary to reach net zero emissions, but is not currently economically viable for power plants to implement at scale. There is some hope that technological developments might bring these costs down.
Capturing carbon at its industrial source is perhaps the best of all solutions if it can be done economically, because fossil fuels are so abundant and affordable and because extensive infrastructure already exists for producing and distributing them. Though considerable additional infrastructure will be needed to transport and store the captured carbon dioxide underground. It is also possible to capture CO2 directly from the atmosphere, but this is currently much more expensive because atmospheric concentrations of the gas are far lower than those at the emissions sources.
Learn More
- MIT Explainers on Carbon Removal Strategies
- Ask MIT Climate: How much carbon would we have to remove to counteract climate change?
- TILclimate Podcast: Today I learned about removing carbon from the atmosphere
- TILclimate Podcast: Today I learned about planting trees
- TILclimate Podcast: Today I learned about carbon capture
10d Adaptation
Whereas the costs of mitigation fall mostly on the largest emitters of greenhouse gases, the costs of adaptation are more broadly distributed over the world. For example, for the past four years, a special government taskforce in Fiji has been trying to work out how to move the country. At present, 42 Fijian villages have been earmarked for potential relocation in the next five to 10 years, owing to the impacts of climate change. At the other extreme, countries such as Russia and Canada might profit from a warmer climate as, for example, melting ice allows for new ocean passageways and uncovers mineral resources. But most nations will need to adapt to climate change, entailing measures ranging from crop substitutions to beefing up seawalls and levees and planning for shifting demands for and supplies of water and food.
A key but complex issue is the relative costs and benefits of adaptation and mitigation, all of which must be estimated in an environment of considerable uncertainty. An optimal strategy will no doubt involve doing some of both.
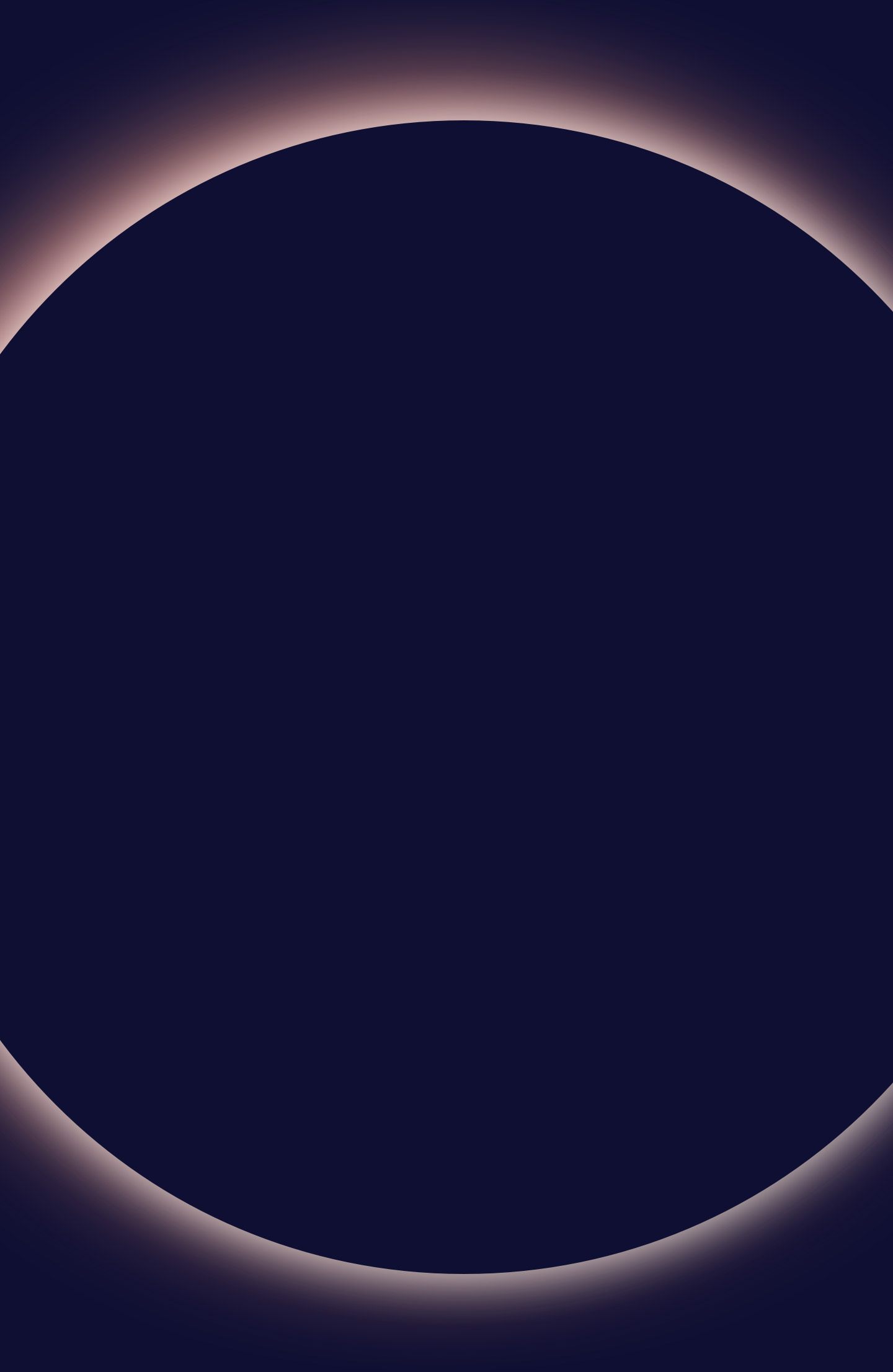
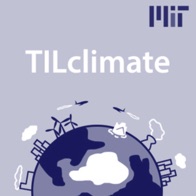
When talking about climate change solutions, we often hear about reducing emissions and adapting to climate impacts, but a third option is starting to get more attention: altering the atmosphere. In this episode, MIT alumnus Janos Pasztor joins host Laur Hesse Fisher to explain geoengineering: what it is and the different technologies that are being researched.
10e Geoengineering
The third approach, geoengineering, seeks to actively counter greenhouse gas-induced warming. Proposals aimed at cooling the earth focus primarily on managing the net amount of solar radiation the planet absorbs by increasing the reflectivity (albedo) of the surface and/or the atmosphere. A popular technique involves injecting modest amounts of sulfur into the stratosphere, resulting in the formation of sulfate aerosols that reflect sunlight and thereby cool the climate system. The technology to do this pretty much exists today, and the cost of doing so is small enough that a small nation or even a wealthy individual could pull it off.
Most of those who work seriously on geoengineering regard it as an option to be kept in our collective back pocket.
But there are many technical, legal, and political problems with solar radiation management. On the technical side, cooling the mean surface temperature back to some desired point (say, enough to prevent damaging sea level rise) while leaving atmospheric concentrations of CO2 unabated would not necessarily repair other important aspects of the climate system. In particular, canceling a long-wave radiative effect (greenhouse gas warming) with a short-wave fix (reflecting solar radiation) does not necessarily restore variables other than temperature. For example, bringing the temperature back to some desired level would almost certainly result in a reduction of global precipitation.
Moreover, engineering solar radiation does nothing to address the CO2-induced acidification of the oceans that may prove to be among the most serious consequences of greenhouse gas emissions.
Furthermore, any entity, whether an individual or a nation, that undertook geoengineering would do so within a largely undeveloped legal framework, leaving it exposed to legal or even military action. For all these reasons, most of those who work seriously on geoengineering regard it as an option to be developed and then kept in our collective back pocket, to be used only if the effects of climate change become catastrophic.
Chapter 11
The Bottom Line
Those nations and businesses that develop economical carbon-free energy early and well will gain an important competitive advantage in what is currently an $8 trillion energy market.
There is overwhelming scientific evidence that the majority of the rapid warming of our planet over the past century has been forced by increasing greenhouse gas concentrations. The concentration of carbon dioxide—the most important long-lived greenhouse gas—is now greater than it has been for at least 800,000 years, and there are plausible futures in which it will reach values not seen since the Eocene period, 50 million years ago. Pushing the climate system this hard and this fast entails serious risks to human civilization, engendered in rising sea levels and associated incidence of storm-related coastal flooding, decreasing habitability of tropical and arid regions, increasing acidification of ocean waters and associated risks to marine ecosystems, and destabilization of the The continuous movement of water on, above and below the surface of the earth.hydrologic cycle with attendant increases in food and water shortages. The latter is especially worrying because of the propensity for past fluctuations in food and water supplies to drive civilizational collapse, rapid migrations, and armed conflict.
While climate science is increasingly confident in its attribution of recent climate change to human-caused changes in greenhouse gases and aerosols, remaining uncertainties in climate physics and climate models lead to large uncertainty in climate projections, with possible outcomes ranging from the challenging to the catastrophic.
There is no scientific justification for the confidence expressed by some that climate change entails little or no risk.
The science suggests that the sooner and more decisively we act to remove carbon emissions from our world’s energy supply, the more damages we’ll avoid. While great progress has been made, the rate of reduction of emissions still isn’t anywhere fast enough to save us from the greatest risks. And there are many ways we can do this. We can scale carbon-free energy sources, technology for capturing CO2 from power plants and industry, and ways to extract CO2 directly from the atmosphere. Renewable energy can power a significant amount of current energy needs, and more if better, more cost-effective energy-storage technology is invented. Nuclear fission has improved remarkably since the 1960s and, once developed, can be ramped up to meet a large fraction of demand in less than 15 years. There is also renewed optimism that nuclear fusion, a basically limitless clean source of energy, may become commercially viable in 10 to 20 years. While this may be too late to significantly curtail major climate risk, it does provide an ultimate target for clean-energy production.
At the present rate of consumption, oil and gas reserves are projected to be exhausted by late in this century, and coal early in the next. Thus, in the not-too-distant future, fossil fuels will have to be replaced anyway. To mitigate climate risk that transition would need to be advanced by several decades. Other countries, notably China, are investing in advanced carbon-free energy sources, including nuclear fission. Those nations and businesses that develop economical carbon-free energy early and well will gain an important competitive advantage in what is currently an $8 trillion energy market.